The Darkness at the End of the Tunnel
A mile beneath the Black Hills of South Dakota, a global physics experiment may shed light on one of nature’s murkiest mysteries.
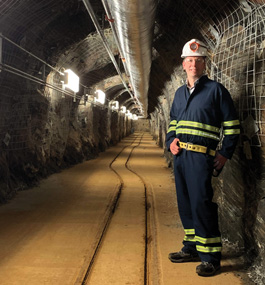
Courtesy SURF
MINE OVER MATTER: Bjoern Penning 4,850 feet underground, in the shaft that leads to the experiment.
by Lawrence Goodman
The Cage, as the elevator is called, leaves at exactly 7:30 a.m. Latecomers are out of luck.
Nearly two dozen people in coveralls, hard hats and thick rubber boots pack inside the Cage before the heavy yellow metal doors are yanked shut and the slow descent into darkness begins. A steady stream of water rains down on them from the timber planks that buttress the elevator shaft, which must be kept continuously wet to prevent rot. Nobody seems to mind. The talk is about family life, weekend plans and what’s for lunch.
About 10 minutes later, nearly a mile down, the lift thumps to a stop. When the doors open, you step into a cavern with rough rock walls.
Until 2002, this was a working gold mine in South Dakota’s Black Hills. Miners once blasted the rock walls with explosives. The rail tracks underfoot carried carts laden with supplies up to the surface. Now they’re being used to send mini-trains with equipment and personnel deep into the tunnels that stretch in every direction.
A little way down one corridor is a clean room where you must change your coveralls, wash your boots and clean your possessions with rubbing alcohol. As you walk farther, it starts to feel more like a regular — though windowless — workplace. Tubing runs overhead and along the walls. Desks press up against one side of the corridor. There’s even an espresso machine and a panini maker.
At the end of the hallway, a pair of doors swing open to reveal a scientific laboratory, the Davis Campus at the Sanford Underground Research Facility. It’s named for Ray Davis, the first physicist to experimentally detect neutrinos emitted from the sun. In the 1960s, while the mine was still a mine, Davis carried out his groundbreaking work down here. Today, the space resembles a villain’s lair in an old James Bond movie. Researchers rush around, checking equipment and monitors. Computers stacked on top of one another hum.
This is where Brandeis physicist Bjoern Penning and his lab, along with 250 other researchers from around the globe, are searching for the ultimate treasure in particle physics — dark matter. One of the most elusive yet ubiquitous substances in the universe, dark matter remains one of the great scientific mysteries.
But Penning and his fellow researchers may be about to crack it.
Do WIMPS rule the universe?
In the 1920s, working atop Southern California’s Mount Wilson, using what was then the world’s most powerful telescope, Caltech astronomer Fritz Zwicky noticed something peculiar about the motion of galaxies hundreds of millions of light-years away.
The stars the Swiss-born Zwicky studied were part of a galaxy group known as the Coma Cluster. The Coma Cluster’s galaxies rotate around its center, much like our solar system’s planets rotate around the sun. Through painstaking work, Zwicky calculated the mass of Coma’s center galaxies to determine the gravitational pull they exerted; the greater the mass, the greater the gravitational pull.
Zwicky soon discovered his numbers didn’t add up. The mass of the center galaxies wasn’t great enough to generate enough gravity to keep the peripheral galaxies in orbit with them. The peripheral galaxies should have broken free from Coma and gone hurtling off into space.
![]() |
‘Just another day at the office’ Watch a video about the search for dark matter a mile underground. |
![]() |
There was only one conclusion. There must be additional mass in the Coma system to keep all the galaxies together, mass that comes not from the stars themselves but from the space between them, hidden from view by the blackness of space. At a 1933 conference, Zwicky theorized this unknown substance was Dunkle Materie, or dark matter.
Zwicky’s theory was promptly forgotten for the next 40 years. Then, in the 1970s, American astronomer Vera Rubin performed calculations similar to Zwicky’s on the Andromeda Galaxy. Rubin, one of the few women in her field, worked at Southern California’s Palomar Observatory (where she had to attach an outline of a skirt to the man icon on a restroom door to create a women’s lavatory). Her results confirmed what Zwicky had found, resuscitating his dark-matter theory.
Subsequent studies gave birth to a new awareness of how little we know about the universe. Atoms, it turns out, account for less than 5% of all matter. Dark matter accounts for 27%. The rest of the universe is composed of an equally mysterious substance called dark energy.
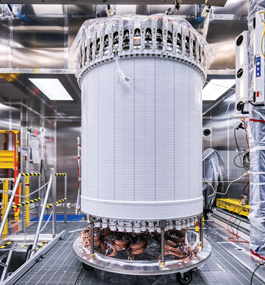
Courtesy SURF
TITANIUM HEART: The dark-matter detector’s innermost chamber, which will eventually be loaded inside the stainless-steel vat and filled with seven tons of liquid xenon.
page 2 of 3
Scientists believe dark matter is most likely made up of subatomic particles called WIMPs, weakly interacting massive particles. WIMPs originated in the early universe along with most other forms of matter, which are made up of particles drawn together by forces like electromagnetism. Unlike those particles, WIMPs are loners. They are chiefly attracted to other particles by gravity, an incredibly weak bond compared to the other forces acting on matter in the universe.
Though WIMPs are all around us, they are not attracted to the atoms that make up our bodies. Like ghosts, billions of WIMPs pass through us every second without our ever knowing.
When WIMPs do crash into an atom, they produce a unique, very faint signal. If the search for dark matter were conducted aboveground, this signal would be drowned out by the cosmic radiation coming from the sun, or being hurled in our direction by collapsing or colliding stars.
This is why SURF’s dark-matter research is being conducted in an abandoned mine beneath the earth. The rock and dirt above reduces the cosmic radiation by a factor of 1 billion. Several other major science experiments are also underway at SURF, which is operated by the South Dakota Science and Technology Authority, and funded by the U.S. Department of Energy, the state of South Dakota and private donations. Despite all the dirt, dust and chipping rock, this abandoned mine is, from a particle physicist’s point of view, an ideally “clean” environment in which to carry out research.
Eliminating the impossible
Penning, 41, joined Brandeis in 2017. He grew up in Spaichingen, a small town in southern Germany at the edge of the Black Forest. At age 7, he received a telescope for Christmas, aimed it at the stars and was hooked. A passion for “Star Trek” naturally followed. “Since I knew I couldn’t become a Starfleet captain,” he says, “I had to do what Spock does — science officer.”
At the nearby University of Freiburg, he studied particle physics as both an undergraduate and a PhD student. His research took him to Illinois to study at Fermilab, the premier particle accelerator in the U.S., where atoms are smashed together at close to the speed of light so scientists can analyze the debris. There, he met his wife, Marcelle Soares-Santos, who, like Penning, is now an assistant professor of physics at Brandeis.
As a faculty member at England’s University of Bristol in the mid-2010s, Penning worked at Switzerland’s Large Hadron Collider, an even bigger particle accelerator than Fermilab. He was part of the team that in 2012 confirmed the existence of the Higgs boson, the particle that gives all other particles mass. It was a major breakthrough: The Higgs was the last undiscovered subatomic particle in the so-called Standard Model of particle physics, which, finalized in the 1970s, is the most complete model to date of how the universe works.
But although the Standard Model encompasses 17 different particles, including quarks, leptons and neutrinos, it does not include WIMPs. When the Large Hadron Collider was built 11 years ago, scientists hoped it would yield evidence of particles outside the Standard Model. It hasn’t, prompting some scientists to doubt WIMPs exist and, instead, to talk about alternatives like axions, sterile neutrinos and WIMPzillas.
In 2013, researchers announced the results of their first attempt to find dark matter. The Large Underground Xenon dark-matter experiment, as it was called, ran for three and a half years. It came up with nothing.
Since then, Penning and researchers from universities and laboratories around the world have revised their design and developed a new detector, the LUX-ZEPLIN, almost 1,000 times more sensitive than the LUX. Penning says it has a much better chance of success.
The LUX-ZEPLIN detector consists of a series of nested sieves, each designed to filter out various subatomic particles so that, at least in theory, any particle that makes it to the center is a WIMP. To underscore the logic, Penning quotes Sherlock Holmes: “When you have eliminated the impossible, whatever remains, however improbable, must be the truth.”
Still under construction, the outermost sieve is a 26-foot stainless-steel vat. Because water blocks the passage of gamma radiation and neutrons, 70,000 gallons of ultra-pure water will be poured inside the vat to keep these particles from progressing toward the interior.
A second sieve will block neutrons, which are a special problem since they induce a weak signal that can easily be mistaken for WIMPs. This sieve consists of 10 12-foot acrylic tanks suspended in the water and filled with liquified gadolinium — neutrons stick to gadolinium atoms — and linear alkylbenzene, a common component of cleaning products.
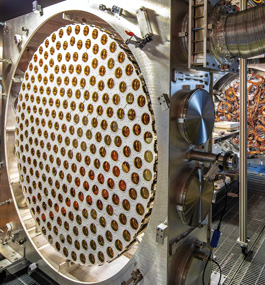
Courtesy SURF
SHARP EYES: Arrays of photomultiplier tubes, ultra-sensitive light sensors that can detect the photons emitted when particles interact with the detector’s sieves.
page 3 of 3
Penning’s team designed the sensors that surround the acrylic tanks. They look like giant K-Cups sheathed in white Tyvek. When neutrons come into contact with gadolinium atoms and get “captured,” photons are emitted. The sensors detect these photons, which signals that everything is working as planned and no neutrons are slipping through the gadolinium barrier.
The innermost sanctum of the experiment — the pièce de résistance — is a 13-foot titanium cylinder filled with liquid xenon. Submerged in the water, the cylinder will be encircled by the acrylic tanks.
If scientists’ theories about WIMPs are correct, then xenon is the planetary element best able to detect dark-matter particles. Densely packed together, xenon atoms can ensnare WIMPs, releasing two flashes of light detectable by sensors in the titanium cylinder to let researchers know dark matter has been found.
Like building a ship inside a bottle
In March 2019, Penning and his lab were at SURF working inside the detector’s stainless-steel container, which was empty except for the titanium cylinder that will eventually hold the xenon. The Penning crew — postdoctoral fellow Ryan Wang, senior mechanical engineer Andrei Dushkin, graduate student Luke Korley and electrical engineer Richard Studley — are building the scaffolding that will run around the inner wall and hold the K-Cup-like sensors Penning designed.
The Brandeis scientists have done only a trial run using dummy parts and equipment. When the detector is turned on later this year, all the other universities collaborating on the experiment will have completed their portion of the installation process inside the container. The Brandeis crew, the last to go, will have only 3.5 feet between the wall and the other scientists’ apparatus in which to work. Penning likens it to building a ship in a bottle while inside the bottle. It requires lots of practice.
The strict cleanliness standards that must be observed make the task especially difficult. WIMPs are so weak that even a speck of dust can obscure their signal and throw off the sensors. If Penning’s team so much as drops a tool or lets a screw fall, the floor could easily be chipped.
So the Penning lab works with a precise rhythm. Dushkin goes up and down a ladder, bolting together the scaffolding’s metal rods. Korley hands him the tools he needs. Studley kneels on the floor, using a laser level to ensure the struts align. They don’t talk much. They know exactly what they need to do.
Meanwhile, Studley is also working on a problem the group will face in the days before the detector becomes operational. They will need to bring a ladder inside with them to erect the scaffolding. They will work their way around the cylinder until they arrive back at the entrance, a small 3-foot-wide portal. But there won’t be enough room to push the ladder out of the portal. The only solution is a custom-built ladder that’s foldable or capable of being disassembled. Studley says it’s doable, but he hasn’t quite figured it out yet.
Toward the end of the day, everyone begins to notice a foul stench, like rotting cabbages or smelly socks. Penning says it’s as if a giant broke wind.
In fact, it’s an evacuation drill. Some of the tunnels where scientists work lack electricity or cellphone reception. The only way to reach them is to release stench gas, which is natural gas with nontoxic levels of the chemical ethyl mercaptan. There are other ways the facility alerts people — alarms, emails and texts — but the stench gas adds an ingenious extra level of safety. Even if you didn’t know it was meant to trigger an evacuation, you’d be desperate to get out.
The stench gas means work must end for the day. The Cage makes only one return trip in the afternoon. Today’s will have to be early. The scientists crowd inside and are carried back to the surface.
When SURF’s dark-matter detector becomes operational, its hundreds of sensors will collect millions of pieces of data every second, seven days a week, 24 hours a day, for the next five years. The scientists will monitor the results on their computers back at their universities.
If a WIMP is discovered, no alarms will sound, no bells will ring. The researchers will simply notice a cluster of dots on a scatter plot. The results will be pored over, checked, double-checked and reviewed by some of the project’s toughest skeptics. Should everything pan out, our understanding of the universe will forever be transformed.
Ray Davis, who did his work deep inside the same tunnel that now houses SURF, went on to win the Nobel Prize in Physics. If Penning and his colleagues succeed in their effort, they could strike the same gold.
SURF operations are on hold due to the COVID-19 pandemic. Scientists expect the experiment to restart later this summer.